QCD, the theory of strong interactions between quarks and gluons, is the "boring" part of the physics of high-energy hadron-hadron collisions. It used to be more more exciting twenty years ago, when the theoretical calculations were not as refined as they are now, and there was still a lot to understand in the physics of strong interactions between quarks and gluons. But nowadays, things are much more clear.
We think we know rather well what happens when quarks and gluons collide, recombine, and produce the streams of collimated hadrons we call "jets". Nowadays the Tevatron experiments, as well as the LHC ones, are focusing on other, more "frontier" physics topics: the discovery of what breaks electroweak symmetry, what gives mass to particles, and whatever lays behind the mask that Nature has put on, the Standard Model.
CDF and DZERO, the two experiments collecting the data from proton-antiproton collisions at the Tevatron 2-TeV accelerator, are undermanned: despite the author lists of the scientific publications they produce indicate that they are still both over 500-strong, much smaller is the number of physicists that are actively producing scientific results. Many former collaborators have left for the LHC experiment, others have left for other endeavours because the Tevatron future was not well known. Some of those who have left, like myself, still appear as authors, for several reasons which would deserve a separate post (ahem).
The shrinking crews of CDF and DZERO have been directed to reinforce the lines of a few very important analyses: those which can still make a difference, with respect to the already huge advance in human knowledge that the experiments at the Tevatron have amassed so far. The hunt for a Higgs boson, the search for supersymmetric particles and other exotic processes, a precision measurement of the top quark mass which the LHC experimenters will sweat department stores of shirts to beat.
Because of that, one does not see too many new QCD results coming out of the Tevatron these days. But the three new results from DZERO are a breath of fresh air.
I might be sounding as if I am a QCD aficionado, but I am not really one. Until yesterday I had never produced analysis results on quantum chromodynamics in my 18-year-long career in experimental high-energy physics. Strange as it may sound, just today, for the first time, I defended at a pre-approval meeting a new analysis of QCD physics. So far, I had done that for Electroweak analyses, Higgs physics analyses, top quark analyses, but no QCD yet. Maybe it is because of the pre-approval that I decided to write about these new QCD results today!
The three results
Too much talking. Let us come to the new QCD results by DZERO. The first analysis producd a measurement of the rate of events with three jets, as a function of their combined invariant mass.
Events with three jets arise when two quarks or gluons collide, scattering one against the other, and one of them radiates a gluon upon leaving the scene of the hard interaction. Indeed, three-jet events were used as the proof for the existence of the gluon in the seventies. Nowadays, they are interesting for another reason: they might hide the production of new processes.
Take for instance a new heavy particle that may be produced in energetic collisions at the Tevatron, and that decays strongly. It might yield three energetic quarks, which would produce three jets. By reconstructing the total invariant mass of the trio, DZERO might find that one certain value appears more often than it should: a peak in the invariant mass distribution signals the creation of a "resonance" - the new particle.
Finding a new jet-decaying resonance would be too good. The reason for precisely measuring the rate of three-jet events as a function of the total mass is a different one, in fact. This is a process that can be calculated with the most up-to-date theoretical tools, and a high-statistics comparison sheds light on the small discrepancies that still exist between experiment and theoretical modeling.
In the figure below, you see that the rate of three-jet events decreases smoothly as a function of mass. There are three sets of black points, which represent the rate of events for three different angular regions. What matters, however, is that theoretical predictions (the blue curves) are right on the money in all cases. Also note how this measurement amazingly extends well beyond invariant masses of one TeV! These are the highest-energy interactions ever recorded so far.
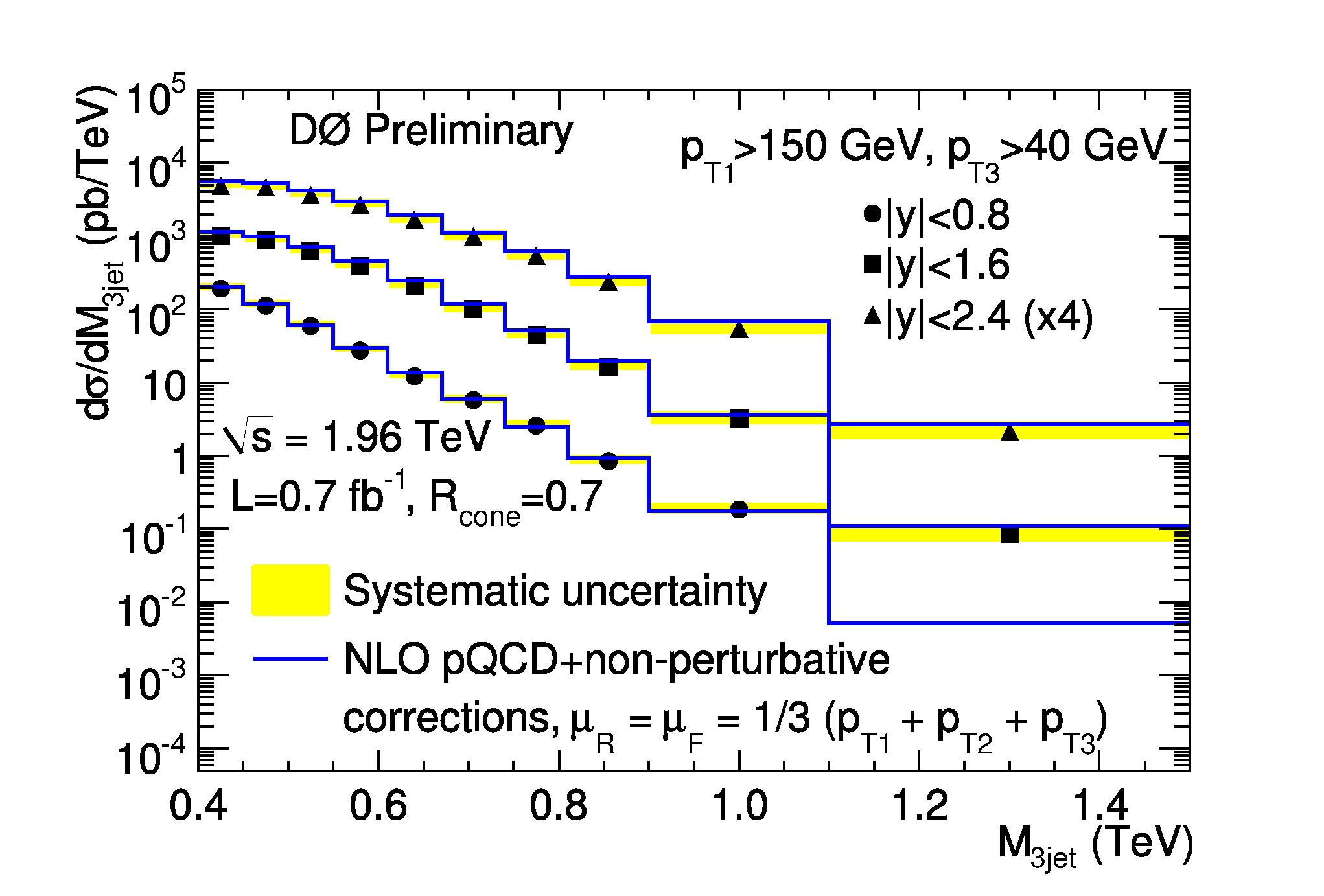
Let us take the second analysis produced by DZERO now. This uses the number of events with three jets and the number of events with two jets to compute a ratio, R3/2, as a function of the energy of the most energetic of them. This ratio is studied for different values of the minimum energy of the other jets. But what is this all about ?
The trick of taking ratios between the rate of different processes is widely used in analyses that wish to remove as many systematic uncertainties as possible. If the numerator has a uncertainty due, say, to the integrated luminosity of the sample used to collect the data, and the denominator has the same uncertainty coming from the same source, the ratio has none: this allows for much more precise comparisons with theory.
The three plots below show the ratio R3/2 computed by DZERO for three different thresholds on the minimum jet transverse energy, 50, 70, and 90 GeV. The data points (black) are compared to several theoretical models, and they unmistakably favor one of them, the Sherpa Monte Carlo. This is quite interesting, because its antagonist Pythia is widely used by most particle physics experiments nowadays, included, of course, DZERO itself. Pythia does a good job only if a particular setting of its parameters, called "BW", is used. Way too technical for us, but the message is clear: this measurement constrains the parametrizations of Quantum Chromodynamical processes in the simulations quite significantly.
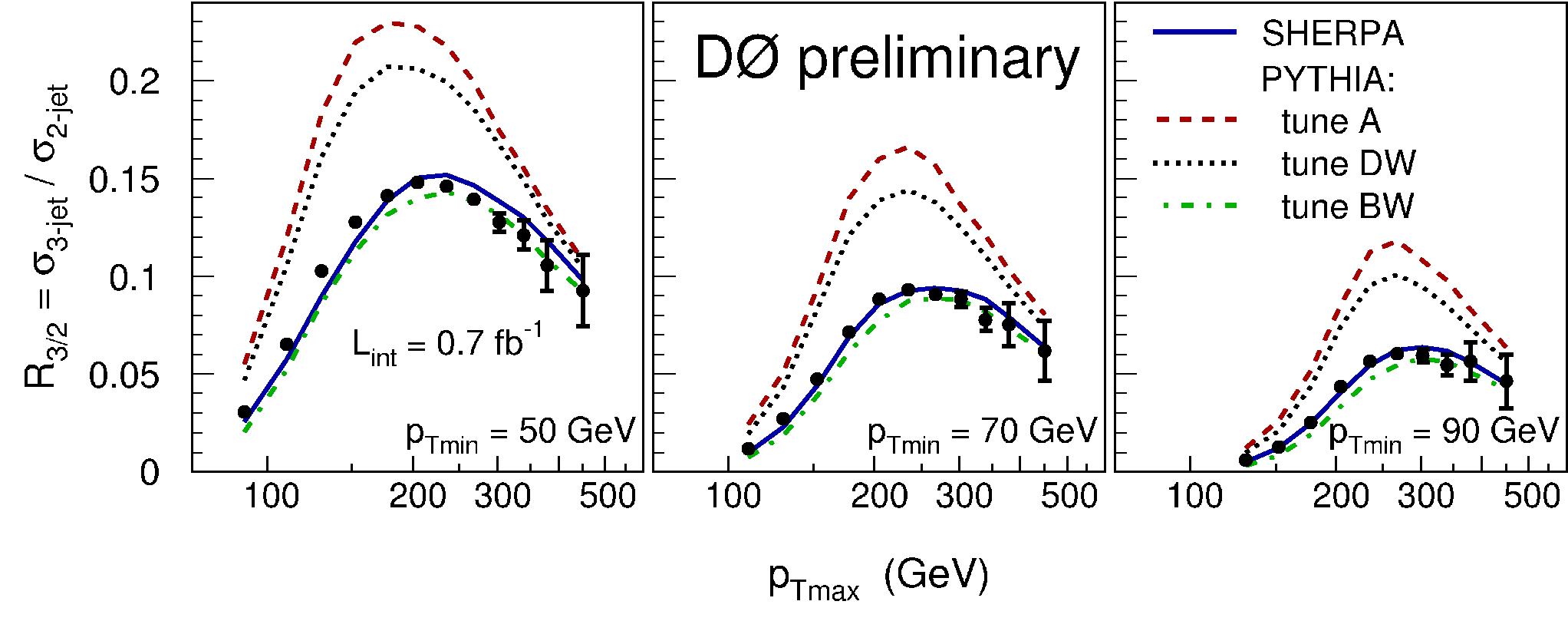
Finally, the third analysis is the most interesting of the three. It reports on a search for exclusive dijet production. Jet pairs are produced "exclusively" when they are the only thing that is observable in the event: this is a quite rare outcome for a energetic proton-antiproton collision, when besides the two interacting quarks or gluons that give rise to the hard jets one has to cope with the remainder of the two colliding particles, which produce a mess of hadrons a bit everywhere in the detector.

You might well then ask: where does the energy of the two jets come from, if the proton and the antiproton remain unscathed ? Well, the proton and the antiproton do retain their identity, but they "slow down": they change direction slightly, yielding part of their energy to the scattering gluons.
In general, these processes where the proton and the antiproton scatter without exchanging color quantum numbers are called "diffractive". Hard diffractive events, such as those searched by DZERO in this analysis, have been observed in a number of other experiments; but hard diffractive events which are exclusive, i.e. where all the energy is yielded to the two final state jets such as in this case, or to another well-defined object, are much harder to observe.
To search for exclusive dijet events, DZERO used a variable which pictures the energy flow in the regions close to the beam axis. It would take too long to explain in detail the discrimination between simple diffractive events and exclusive ones; suffices to say that the variable Delta takes on values close to unity only for the exclusive processes, which are extremely rare and well hidden otherwise.

You can see how Delta discriminates the exclusive events, as predicted by the simulation, in the figure above. The data (black points) are compared to the distributions expected from non diffractive (NDF), single diffractive (SD), double diffractive (IDP), and exclusive diffractive processes (EDP). In the last bin, exclusive events are the largest contribution.

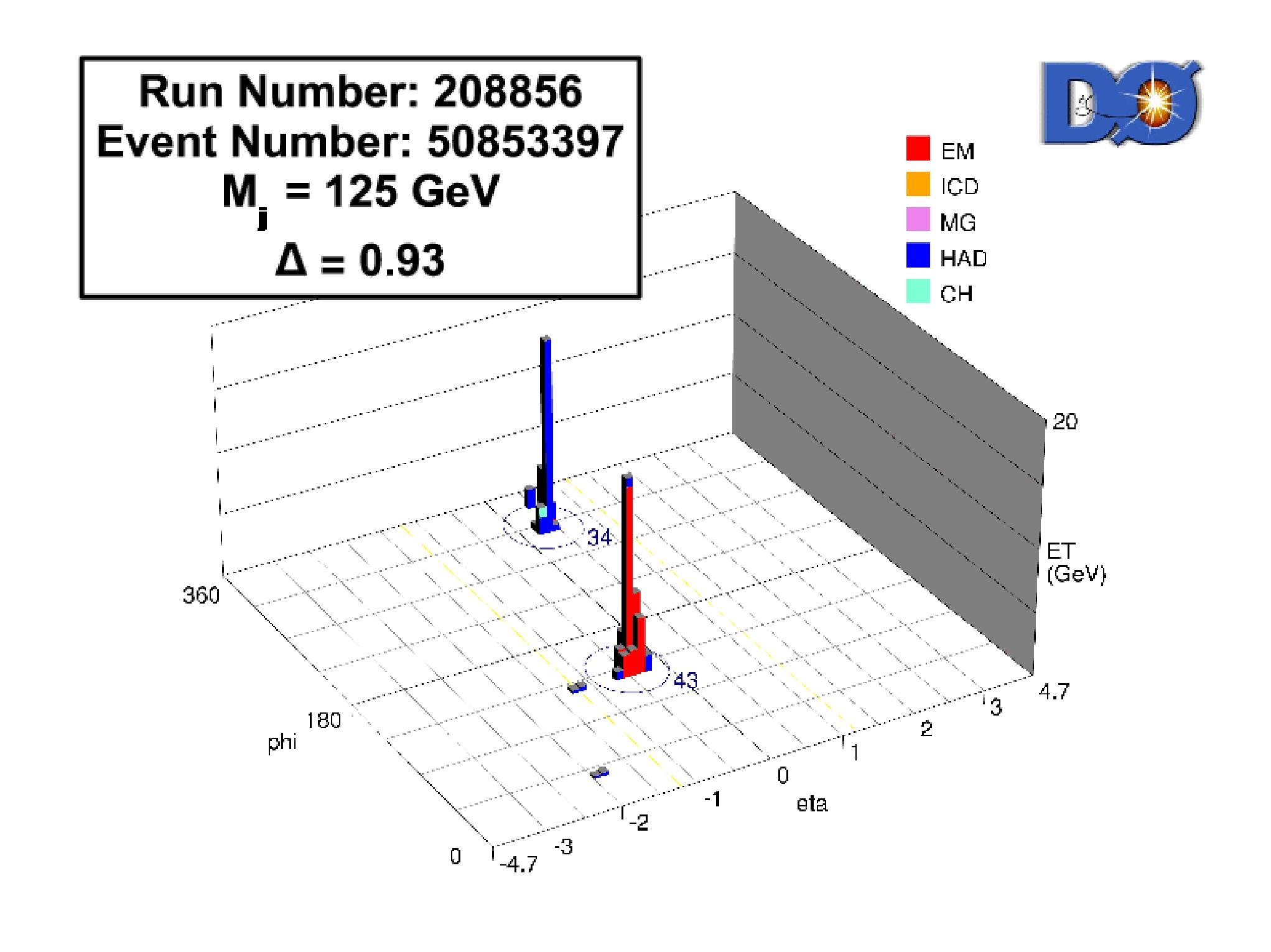
Above you can see an exclusive dijet production candidate isolated by DZERO. Quite nice! There indeed is nothing in the event but two well-separated, back-to-back streams of hadrons. The graph, if you are unfamiliar with these kinds of "lego plots", pictures the energy deposits in the DZERO calorimeter. Higher bars represent larger energy deposits. The calorimeter may be thought as a cylinder surrounding the beam axis around the point where the particles collide: the cylinder is cut along its height, unrolled, and the lego plot is drawn on top of its surface.
In conclusion, three new results by the DZERO experiment advance significantly our understanding of quantum chromodynamics in the high-energy regime (three-jet masses above 1 TeV), in the subtleties of the three-jet ratio (simulation tunings necessary to get in agreement with the data), and in the extremely-low-rate phenomena of exclusive diffraction. It only remains to present my congratulations to the DZERO scientists for these important achievements!
Comments