So with two giant underground detectors (a near and a far unit, defined by their distance from Fermilab, where is located the particle accelerator which provides the studied neutrinos) what can you do, except looking for the elusive signal of neutrino interactions ? Of course, you can study cosmic rays.
Cosmic rays are particles -mostly protons and light atomic nuclei- that originate in very energetic phenomena in the cosmos. When they impinge on the Earth's atmosphere, these particles may interact with oxygen or nitrogen nuclei, creating a shower of secondary particles. At sea level we are bombarded by a mixture of these secondary products: protons and electrons, which can be shielded by concrete or heavier material, and muons, which are very penetrating.
It is precisely muons that underground detectors like the MINOS near and far detector are more sensitive to: in fact, neutrino detectors are placed under as much ground and rock as possible exactly for the purpose of reducing background from cosmic rays. Neutrino physicists would be happy to suppress all of that background; but as muons cannot be stopped easily, and still shower the detectors with a measurable flux, well then why not study them ?
Muons in cosmic rays mostly originate from the decay in flight of kaons and pions; those are in turn produced by the hadronic showers created by the hard interaction of the incoming proton or light nucleus with the oxygen or nitrogen nucleus of the upper atmosphere. These reactions are quite well understood at particle physics level. So one might think there is not much to learn from them.
The fun begins once one realizes that the simple muon counting rate (the number of muons that cross your detector in unit time) already yields interesting information: for starters, it is not constant over time, as it exhibits a seasonal variation. In the summer the atmosphere is hotter and thus less dense, so secondary pions and kaons have a smaller probability of interacting with an oxygen or nitrogen nucleus. This leaves them more time to decay into muons, and you thus observe an increased muon flux on the ground. Hence your detector can be used to know what's the weather like outside! Is that cool or not ?

The MINOS data becomes more interesting if one studies the rate at which two or more muons are simultaneously detected. Given the excellent timing resolution of the detector, these multiplets are usually coming from the same primary cosmic ray interaction, via the decay of different secondary pions or kaons (energetic cosmic showers may yield thousands of such particles). Surely, you would expect that multiple-muon events would also exhibit the same seasonal variation, right ?
That's not what MINOS finds. In fact, they find an opposite trend in the rate of multiple-muon events, as shown in the graph below. So that's a puzzle waiting to be solved.
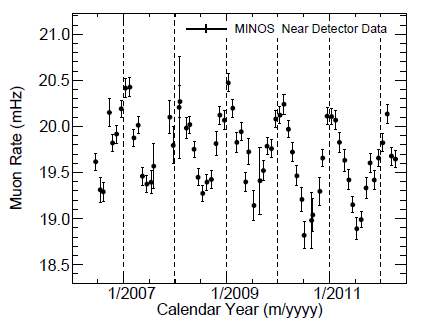
There are four possible explanations put forth in the article describing these observations. They involve geometric effects connected with the spread of the muons (in the summer they would spread further apart due to atmospheric density effects, yielding fewer "multi-muon" events in the detector), or a rise in the fraction of dimuon decays due to the larger number of such hadronic resonances produced when pions or kaons have more time to interact, or altitude-dependent temperature effects. It is actually quite fun to try and solve this riddle, and it may give us more insight in the detailed properties of atmospheric showers from cosmic rays. Their precise modeling is very useful in the study of ultra-energetic cosmic rays, which are a much bigger mystery.
So you see, doing science is really about studying whatever there is to study, with whatever tools you have handy. If you do it with care, you are guaranteed to learn something more about the world around you.
Comments