The cytoplasm of mammalian cells is a viscous fluid, with organelles and proteins jiggling against one another and drifting at random.
Yet a new biophysical study finds that those drifting objects are subject to a very different type of environment than what we have thought.
The cytoplasm is actually an elastic gel, it turns out, so it puts up some resistance to simple diffusion. But energetic processes elsewhere in the cell—in the cytoskeleton, especially—create random but powerful waves in the cytoplasm, pushing on proteins and organelles alike. Like flotsam and jetsam buffeted by the wakes of passing ships, suspended particles scatter much more quickly and widely than they would in a calm sea.
Because transport within the cytoplasm therefore depends mainly on separate processes that consume energy, a measurement of the spectrum of forces exerted on the cytoplasm at any given time can provide a snapshot of the metabolic state of the cell.
Within the cytoplasm, fluctuating forces enhance the intracellular transport of proteins and organelles. Image courtesy of Ming Guo, Harvard SEAS.
Led by David A. Weitz, Mallinckrodt Professor of Physics and Applied Physics at the Harvard School of Engineering and Applied Sciences (SEAS), a team of applied physicists and cell biologists have put forth this new model of the cytoplasm and demonstrated a way to quantify the aggregate forces felt by particles and organelles in the cell. Their findings, published online August 14 in the journal Cell, raise a host of new questions about cellular dynamics. They also provide a robust new tool for future investigations.
"Our research provides the first real physical understanding of the cytoplasm in mammalian cells," says lead author Ming Guo, Ph.D. '14, a former graduate student in applied physics at Harvard SEAS who is now continuing as a postdoctoral fellow to further explore the fundamental biophysics of cells. "This work is going to be critical for future research on development, cancer biology, and metabolism."
Until now, cell biologists have assumed that particles and other objects passively diffuse through the cytoplasm because they collide randomly with neighboring molecules—a process called thermal diffusion or Brownian motion. After all, under a microscope, the jerky movements of such particles do resemble thermal diffusion. Relying on this assumption, and lacking the tools to test it, Guo says, researchers in the field have underestimated the importance of the cytoplasm as a participant in more complex cell dynamics.
"I talked to many people at different universities, and it seems a lot of them think, 'Oh, this is very straightforward—this should just be thermal diffusion,'" he explains. "It's such a beautiful idea. It's very simple. It makes perfect sense. But it's just not the case."
Just as a spoon can stir up sugar in a coffee cup, helping it to dissolve, the operations of cellular machinery prevent suspended particles from settling into equilibrium. Molecular motors that repeatedly tug on strings of actomyosin, the building blocks of muscle fibers, are the main culprit, but other enzymatic activities can also create these waves.
Guo, Weitz, and their collaborators tested this theory through a series of "knockout" experiments in which they removed the cells' fuel source, adenosine triphosphate (ATP). In the starved cells, suspended particles and endogenous organelles traveled far more slowly.
They also developed a new procedure for measuring the overall magnitude of the fluctuating forces within the cytoplasm of live cells, as well as the dependence of these forces on frequency. They call it force spectrum microscopy (FSM), because it uses a combination of microscopy, microrheology, and optical tweezers to measure the stiffness of the cytoplasm and the movement of an injected, inert particle. The difference between the observed movement and the expected thermal movement from the measured cytoplasmic mechanics provides enough information for them to calculate the other forces within the cell.
"This is the first time it has been possible to measure the aggregate forces in a cell, which now will allow us to distinguish the state of its motor or enzymatic activity under different conditions or subjected to different stimuli," says Weitz. "That information should provide a completely new perspective on cell behavior."
In addition to his role at Harvard SEAS, Weitz is the director of Harvard's Materials Research Science and Engineering Center, co-director of the BASF Advanced Research Initiative, a member of the Kavli Institute for Bionano Science&Technology, and an associate of the Wyss Institute for Biologically Inspired Engineering.
The jerky movement of a particle within the cytoplasm does resemble thermal diffusion, 'but it's just not the case,' says Guo. Image courtesy of Ming Guo, Harvard SEAS.
"Our results imply that factors affecting the activities of molecular motors indirectly orchestrate the overall rheology of the cytoplasm," says coauthor Jennifer Lippincott-Schwartz, Distinguished NIH Investigator and Chief of the Section on Organelle Biology at the Eunice Kennedy Shriver National Institute of Child Health and Human Development (NICHD).
"The conclusion that the cytoplasm is best modeled as an elastic gel, out of equilibrium, rather than as a Brownian-based fluid has important implications," she says. "It means that factors modulating the stiffness and motion of the cytoplasm derive from the cell's underlying energetic state."
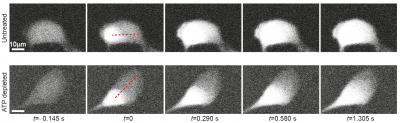
In comparison to those in normal cells (top row), fluorescent proteins in ATP-depleted cells (bottom row) took much longer to diffuse from one side of a cell to another—the distance indicated by the red dotted line. According to the study in Cell, ATP-driven processes produce the fluctuating forces required for proper transport within the cytoplasm. Image courtesy of Ming Guo, Harvard SEAS.
The research team observed this in practice when they measured and compared the cytoplasmic forces within benign and malignant breast cells. The hyperactive malignant cells exhibited much stronger aggregate forces.
In short, changes in the activities of molecular motors and other enzymatic activity can affect cellular properties like the stiffness of the cytoplasm and how easily objects can move within it. Cytoplasmic changes might then affect other cellular activities, further downstream. Future studies using force spectrum microscopy may shed new light on the elastic properties of both the cytoplasm and the nucleoplasm, and how these properties affect gene expression, metabolic signaling, cell growth, and motility.
Comments