In the past 2 million years, three eruptions at Yellowstone have spewed enough volcanic ash to cover half of North America. The new study doesn't speculate about the chances of another cataclysmic caldera eruption at Yellowstone but it gives new perspective on the deep reservoir of fiery material that feeds eruptions.
In a December 2009 analysis, Robert B. Smith, professor emeritus and research professor of geophysics at the University of Utah and a coordinating scientist of the Yellowstone Volcano Observatory, used seismic waves from earthquakes to make the most detailed seismic images yet of the hotspot plumbing that feeds the Yellowstone volcano. Seismic waves move faster through cold rock and slower through hot rock. Measurements of seismic-wave speeds were used to make a three-dimensional picture, like X-rays are combined to make a medical CT scan.
The 2009 images showed the plume of hot and molten rock dips downward from Yellowstone at an angle of 60 degrees and extends 240 kilometers (150 miles) west-northwest to a point at least 660 km (410 mi) under the Montana-Idaho border – as far as seismic imaging could 'see.'
In the new study, images of the Yellowstone plume’s electrical conductivity, generated by molten silicate rocks and hot briny water that is naturally present and mixed in partly molten rock, shows the conductive part of the plume dipping more gently, at an angle of perhaps 40 degrees to the west, and extending perhaps 640 km (400 mi) from east to west. The geo-electric image can “see” only 320 km (200 mi) deep.
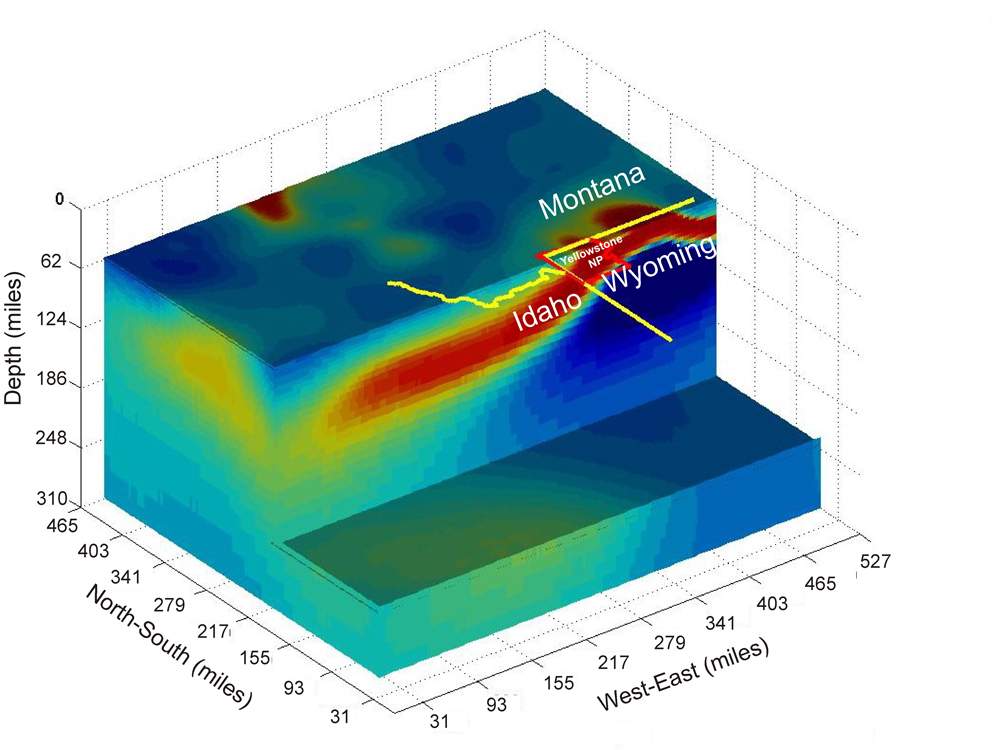
Variations in electrical conductivity of underground rock, shows the volcanic plume of partly molten rock that feeds the Yellowstone supervolcano. Yellow and red indicate higher conductivity, green and blue indicate lower conductivity. Made by University of Utah geophysicists and computer scientists, this is the first large-scale "geo-electric" image of the Yellowstone hotspot. Credit: American Geophysical Union
Smith says the geo-electric and seismic images of the Yellowstone plume look somewhat different because “we are imaging slightly different things.” Seismic images highlight materials such as molten or partly molten rock that slow seismic waves, while the geoelectric image is sensitive to briny fluids that conduct electricity.
“It [the plume] is very conductive compared with the rock around it,” says geophysics Professor Michael Zhdanov of the University of Utah in Salt Lake City, principal author of the new study and an expert on measuring magnetic and electrical fields on Earth’s surface to find oil, gas, minerals and geologic structures underground. “It’s close to seawater in conductivity.”
The lesser tilt of the geoelectric plume image raises the possibility that the seismically imaged plume, shaped somewhat like a tilted tornado, may be enveloped by a broader, underground sheath of partly molten rock and liquids, Zhdanov and Smith say.
“It’s a bigger size” in the geoelectric picture, says Smith. “We can infer there are more fluids” than shown by seismic images. Despite differences, he says, “this body that conducts electricity is in about the same location with similar geometry as the seismically imaged Yellowstone plume.”

Zhdanov says that last year, other researchers presented preliminary findings at a meeting comparing electrical and seismic features under the Yellowstone area, but only to shallow depths and over a smaller area.
The study was conducted by Zhdanov, Smith, two members of Zhdanov’s lab – research geophysicist Alexander Gribenko and geophysics Ph.D. student Marie Green – and computer scientist Martin Cuma of the University of Utah’s Center for High Performance Computing. Funding came from the National Science Foundation (NSF) and the Consortium for Electromagnetic Modeling and Inversion, which Zhdanov heads.
The Yellowstone Hotspot at a Glance
Almost 17 million years ago, the deep plume of hot and partly molten rock known as the Yellowstone hotspot first breached the surface in an eruption near what is now the Oregon-Idaho-Nevada border. As North America drifted slowly southwest over the hotspot, there were more than 140 gargantuan caldera eruptions – the largest kind of eruption known on Earth – along a northeast-trending path that is now Idaho’s Snake River Plain.
The hotspot finally reached Yellowstone about 2 million years ago, yielding three huge caldera eruptions about 2 million, 1.3 million and 642,000 years ago. Two of the eruptions blanketed half of North America with volcanic ash, producing 2,500 times and 1,000 times more ash, respectively, than the 1980 eruption of Mount St. Helens in Washington state. Smaller eruptions occurred at Yellowstone in between the big blasts and as recently as 70,000 years ago.
Seismic and ground-deformation studies previously showed the top of the rising volcanic plume flattens out like a 480-kilometer-wide (300 mile-wide) pancake 80 km (50 mi) beneath Yellowstone. There, giant blobs of hot and partly molten rock break off the top of the plume and slowly rise to feed the magma chamber – a spongy, banana-shaped body of molten and partly molten rock located about 6 km to 16 km (4 to 10 mi) beneath the ground at Yellowstone.
Computing a Geoelectrical Image of Yellowstone’s Hotspot Plume
Zhdanov and colleagues used data collected by EarthScope, an NSF-funded effort to collect seismic, magnetotelluric and geodetic (ground deformation) data to study the structure and evolution of North America. Using the data to image the Yellowstone plume was a computing challenge because so much data was involved.
Inversion is a formal mathematical method used to “extract information about the deep geological structures of the Earth from the magnetic and electrical fields recorded on the ground surface,” Zhdanov says. Inversion also is used to convert measurements of seismic waves at the surface into underground images.
Magnetotelluric measurements record very low frequencies of electromagnetic radiation – about 0.0001 to 0.0664 Hertz – far below the frequencies of radio or TV signals or even electric power lines. This low-frequency, long-wavelength electromagnetic field penetrates some three hundred kilometers (about 200 mi) into the Earth. By comparison, TV and radio waves penetrate only a fraction of a centimeter (inch).
The EarthScope data were collected by 115 stations in Wyoming, Montana and Idaho – the three states straddled by Yellowstone National Park. The stations, which include electric and magnetic field sensors, are operated by Oregon State University for the Incorporated Research Institutions for Seismology, a consortium of universities.
In a supercomputer, a simulation predicts expected electric and magnetic measurements at the surface based on known underground structures. That allows the real surface measurements to be “inverted” to make an image of underground structure.
Zhdanov says it took about 18 hours of supercomputer time to do all the calculations needed to produce the geoelectric plume picture. The supercomputer was the Ember cluster at the University of Utah’s Center for High Performance Computing, says Cuma, the computer scientist.
Ember has 260 nodes, each with 12 CPU (central processing unit) cores, compared with two to four cores commonly found on personal computer, Cuma says. Of the 260 nodes, 64 were used for the Yellowstone study, which he adds is “roughly equivalent to 200 common PCs.” To create the geoelectric image of Yellowstone’s plume required 2 million pixels, or picture elements.
Citation: Michael S. Zhdanov, Robert B. Smith, Alexander Gribenko, Martin Cuma, and Marie Green, 'Three-Dimensional Inversion of Large-Scale EarthScope Magnetotelluric Data Based On The Integral Equation Method: Geoelectrical Imaging Of The Yellowstone Conductive Mantle Plume', Geophysical Research Letters, no publication date provided yet
Comments