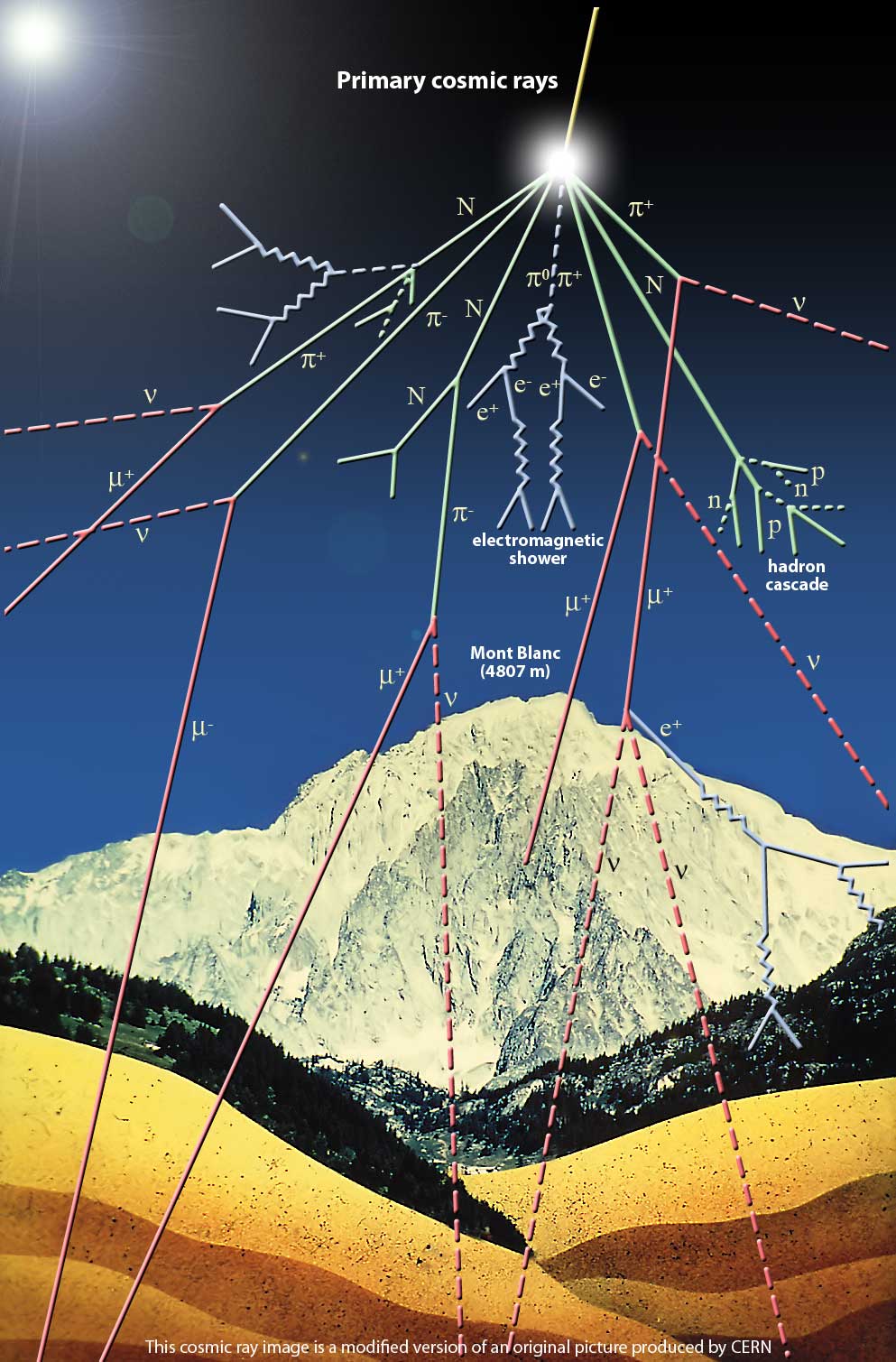
The final result that can be observed on the ground is a shower of "stable" particles: photons, protons and neutrons, electrons, muons, plus some as-yet-undecayed pions and kaons; along with these, of course there remain neutrinos, which escape unseen.
Notice that above I placed the muons in the category of "stable" products, although their lifetime is of the order of 2 microseconds: a 10-GeV muon produced in the decay of a pion 10 kilometers overhead will travel on average 60 kilometers before decaying, and thus most of the energetic muons produced high up will still reach us on the ground.
If you want the details of the calculation, they are quite simple. All we need is the relativistic "gamma factor"

Cosmic rays of the highest energy -we are talking about billions of billion electron-volts, e.g. more than a million times the energy with which protons are accelerated in the core of the Large Hadron Collider at CERN- are very rare, and they have an extra-galactic origin. Particles impinging on Earth with lower energy get deflected by the strong magnetic fields of our galaxy, so their direction of provenance is randomized and does not provide us with information on the sources; but the highest-energy ones do not get deflected, and with them we can study the distribution of the sources, and understand more about a possible correlation in their arrival direction and time with other signals. The author of the paper actually explains this better than I did above, so I advise you to have a look at the paper.


On the horizontal axis you get the primary cosmic ray energy (computed with the help of the detected energy, the shape of the shower, and other information provided by the many detecting elements), in electron-volts (an electron-volt, in case you are wondering, is the energy that an electron acquires by being accelerated by a potential difference of one volt). On the vertical axis you get the cosmic ray flux, in a funny combination of "normal" flux units -per square kilometer, per unit of solid angle, and per year- and "normalization units" -energy to the third power- which are needed to make the spectrum "flatter". In fact, the flux is a steeply falling distribution as a function of the cosmic ray energy, so that if it were plotted as it is it would be hard to see its prominent features.
Leaving alone the above scale trick, we get a feature-full distribution that is quite interesting! There is a dip (called "ankle" by experts) at a few 10^18 eV, the second is the quick cut-off at the highest energies. Let us speak the author:
"The first break is known as the ankle and can be interpreted as marking the transition between galactic and extra-galactic cosmic-ray origins. While lower energy cosmic rays are trapped by the galactic magnetic fields, the high energy flux may be dominated by particles that originated in very distant sources. As the energy increases their trajectories will be less and less affected by magnetic fields, and they will start pointing back to the sources. Astronomy might thus become possible. The second break could be due to the end of accelerating power at source, but it has been predicted long ago that cosmic ray fluxes at these high energies would have to be attenuated by collisions with the Cosmic Microwave Background (CMB). When the center-of-mass energy of those collisions is above roughly 1 GeV/c2, they lead to nuclear disintegration and photo-pion emission from the excitation of proton resonances.All this is extremely interesting. There are many unsolved mysteries in the highest-energy cosmic rays, but we are making giant steps toward their solution. One mystery I did not discuss here is the identity of the primary cosmic ray: for a discussion, I suggest you to browse the paper -it is very well written.
Most nuclei can only travel a few tens of Mpc. Even pure beams of the very stable iron nuclei or proton primaries would be attenuated to 50% after 100 Mpc. The effect described for protons is known as the GZK cut-off.
Most of the high energy cosmic rays detected arrive isotropically, their directions modulated only by the detector exposure. For the highest energy data, however, the arrival directions show a correlation with the locations of the nearest extra-galactic potential sources. An excess of events is seen close to Centaurus A, the closest Active Galaxy Nuclei (AGN): within 18°, 12 events are seen, while only 2.7 were expected from an isotropic distribution."
Comments