The B meson is a very fancy particle: its featured b-quark cannot be found in ordinary matter and is only produced in energetic particle collisions. However, the eight-years-worth of data collected by the Tevatron experiments contain billions of them by now. The CDF and DZERO experiments are thus capable of studying these particles in close detail, with analyses that might be divided in two classes: systematics-limited precision studies of macroscopic characteristics of B mesons, exploiting the detailed features of their most common decays to put the underlying electroweak theory to the test; and statistics-limited investigations of the phenomenology of their rarest decay modes. Both these strategies provide stringent tests of the standard model, and both could in principle reveal telling deviations if some new physics process modified the phenomenology.
While what limits the accuracy of the measurement of generic properties, which can be studied in large-statistics samples, are systematic effects which are usually very hard to impossible to beat down, what determines the sensitivity of a statistics-limited search is the amount of time that one is willing to wait as the detector collects the data. So in a sense the latter is a much "easier" endeavour!
Granted that one cannot say that precision measurements are hard while searches for rare processes are easy -since every data analysis is hard if done outstandingly, or easy if done in a sloppy manner- there is indeed a distinction to make: new physics is expected to stick out more clearly in the phenomenology of rare decays, because what makes these decays rare might just be a standard model rule, one which new physics is immune of. This, in essence, is why we are driven to search for the rarest phenomena: a one-part-per-ten-million effect may increase the rate of a one-in-a-million process by 10%, or the rate of a one-in-ten process by a millionth. Clearly, it is easier to detect the former!
If we look in the PDG -the particle physicist's bible, which collects every measurement of subnuclear particles produced in the last seventy years or so- we find pages and pages and pages of measurements. Behind each line in the PDG there is the dedicated effort of dozens of scientists... Their work is not in vain.
It turns out that some of the best quark transitions to study -those which might in principle be the place where new physics first gives an observable effect- involve the transmutation of a b-quark into a s-quark. Since the s-quark has the same electric charge of the b-quark, the resulting decays proceed through neutral currents. The quark line somehow manages to magically change flavour, and nothing else: the resulting emitted "current" which originates from this transition may then materialize a pair of leptons, allowing us to spot the rare occurrence.
CDF can study the

Of course the annoying constraints mentioned above only affect the standard model processes. New physics might be capable of turning the b-quark directly into a s-quark without an intermediate state; or it might provide an extra boson which couples more readily to these fermionic fields; or it might allow extra particles in the virtual loops that change the quark flavour. All these possibilities would "speed up" the reaction, making it more likely to occur. They might also manifest themselves in modified angular distributions of the decay products, because a different interaction will "kick out" the final state particles in a different way from that expected from standard model interactions.
There is further attractiveness in the above reactions: the final states only contain charged particles -most notably, there are no neutrinos annoyingly carrying away part of the energy balance of the decay; but also no neutral pions. Neutral pions decay immediately after creation into two photons, and these can only be seen by the electromagnetic calorimeter, where they are often lost in the background from other hadrons produced in their proximity. In summary the absence of neutrinos or other neutral particles, which would make the full reconstruction of the initial mass of the decaying particle much harder, is a very welcome characteristics of these rare processes. Note that the K*(892) meson -a neutral particle!- is an excited version of the neutral kaon: it immediately decays into a charged kaon and a charged pion -again, charged particles!
CDF had searched for the three decays above in about one inverse femtobarn of collisions, in 2007, and extracted significant peaks of each B meson; significant, but not yet "observation-level" signals. Time would tell, as I mentioned above. And in fact, by analyzing a dataset almost five times as large, and by refining the analysis strategy, the three signals have finally made it well past the five-sigma mark. Two of them had already been studied at the B factories; but the one involving the B_s meson is new ground!
Below you can see how the three resonances look like. The
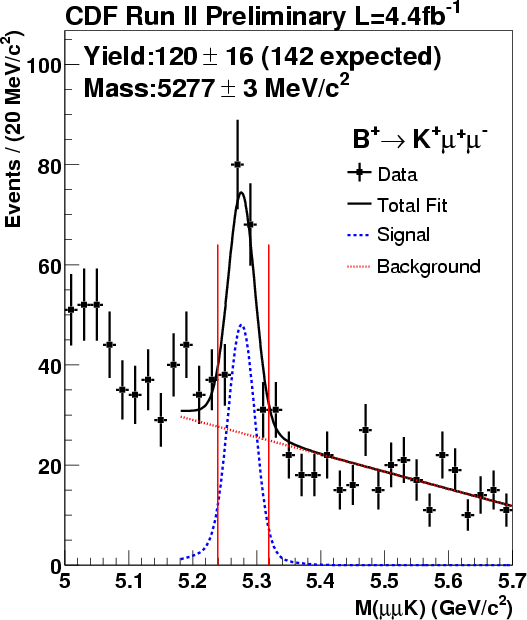
... the
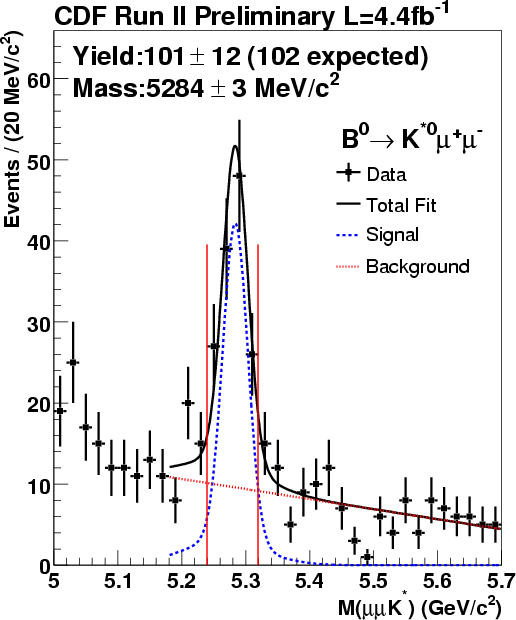
... and the

In all figures, the black points represent the experimental data distribution in the reconstructed mass of the decaying meson; the red hatched line is the background model, the blue hatched line is the signal model, and the black line is the sum of signal and background returned by the fit.
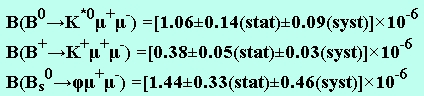
Unfortunately, the measured rates are all in excellent agreement with standard model predictions. So there appear to be no exotic mechanisms that enhance the frequency of such decays. However, it must be said that none of the most en vogue models of new physics are ruled out by these measurements: to really cut into the flesh of supersymmetric theories, for instance, we will need to measure branching fractions of the order of 10^-9, such as in the direct decays
Now, the interesting thing about the new measurements is that despite the rarity of the decays seen, the samples are large enough to allow a first study of some angular distributions, which might be able to discriminate standard model production and new physics models. This might sound silly: if the rate agrees with the standard model, how can the kinematics disagree ? Well, the fact that the rate is okay does not mean by itself that there is no margin for a component of decays due to exotic processes in the data; the statistical error is large enough to prevent very stringent statements. The event shape is always complementary, additional information which may, in some cases, provide the smoking gun for a new discovery even when there are no rate disagreements.
As an example, have a look at the figure shown below. In it, you can see the forward-backward asymmetry of one of the the studied decays, that of the neutral B meson, as a function of the square of the energy released in the particle's disintegration (labeled "q^2" in the horizontal axis).
[If you are very curious about the physics: the forward-backward asymmetry displayed is a quantity one may extract from the distribution of the muons emitted in the decays. It depends by a few so-called "Wilson coefficients", which may be used to describe the kinematics of flavor-changing neutral current processes. In particular, the factor named
The black squares show the asymmetry in five independent bins of q^2, the red curve shows the standard model prediction for the asymmetry, and in blue is shown the distribution one would observe from a new physics model which were to invert the factor

(One detail some of you may be wondering on is the meaning of the green bars, and why there is no data point in those regions of q^2. The answer is that in those regions the production is dominated by the resonant modes involving the exchange of vector mesons, the J/Psi and the Psi(2S). The processes are then different, and not nearly as rare -they are in fact 1000 times more frequent. The q^2 values encompassed by the green bands is the square of those two particle masses).
I look forward to more studies of these rare decays by CDF and DZERO. Bear in mind that these two experiments are expected to produce results with a statistics more than doubled with respect to the one used in this analysis. Twice more data will not change the situation much, admittedly, but it might be enough to spot some interesting deviation from theory. In any case, these rare decays are bound to become business for the Large Hadron Collider, which is expected to collect not twice, but a hundred times more data, at a center-of-mass energy five to seven times higher.
Comments