When I gave a look at the Arxiv this morning and saw a nice new paper by DZERO on the production of single top quarks, I could not help thinking that this is about it, as far as Tevatron single top studies are concerned, speaking of course of DZERO contribution to this particular topic. The analysis uses over five inverse femtobarns of proton-antiproton collisions, and in principle it could soon almost double the statistics with the additional data that will become available after the final shutdown of the machine (the final datasets of CDF and DZERO will total about 10 inverse femtobarns of recorded data); but given the complexity of this analysis and the need of the DZERO physicists to start getting involved in other experiments, I have the feeling that a reanalysis might not happen.
To add interest to the paper, I must explain that the search for single top quark production has been a very long and hard one at the Tevatron in the course of the last decade. While top pair production now seems a commonplace phenomenon in 2-TeV proton-antiproton collisions, with thousand-strong event samples now being used for precise measurements, single top production has been a headache for ten years after the discovery of the sixth quark, and is still a difficult reaction to put in evidence today.
How to produce a single top quark
The production diagrams all involve a W boson in the initial state, and thus a weak production mechanism which makes the process much less likely to occur than strong-interaction processes requiring the same energy; however, since this energy is smaller than that necessary to produce two top quarks (the former being of the order of the mass of one top quark, while the latter being of the order of the mass of two of them), in the end the cross section for producing a single top is in the same ball-park as that for producing a pair: about three picobarns against about eight.
[When I speak of "requiring the same energy" I of course refer to the subprocess energy, i.e. the one available to the reaction among proton and antiproton constituents. At a hadron collider, the total center-of-mass energy is fixed (1.96 TeV in the case of the Tevatron collisions), but the quarks and gluons that take part in the hard subprocess -the ones that do collide- take a fraction of that total energy; that fraction is much smaller, with large fractions being quite rare.]
A three picobarn cross section means that only one signal event is produced if you collide twenty billion proton-antiproton pairs: a mindboggling concept, but at the Tevatron we have observed even rarer processes, so that is not the real issue.
The real problem with extracting the signal of a single top quark in hadronic collisions is not the faintness of the signal by itself, but rather the smallness of the signal to background ratio, which cannot be increased by ordinary means above a certain level. While top quark pairs feature six energetic, usually well-identified objects in the final state (three from each top), single top quarks usually feature four. Let us see where these four objects arise from.
One possibility is that a quark-antiquark pair (note that these need to be quarks of different flavour!) "fuse together" creating a W boson. If the quark-antiquark system has a total center-of-mass energy exceeding twice the W mass, the W will be way "off mass shell", i.e. it will be a very fat guy. Unlike a regular-weight W, this one will be capable of decaying into a top-antibottom quark pair, a process which a regular W could not produce because of energy conservation.
It is easy to see why that is so: the top quark weighs 173 GeV, and the bottom quark weighs 4 more, so the W must carry a rest mass of at least 177 GeV. Such virtual W bosons do exist, as the graph on the right shows are indeed detected (the W bosons resulting in the figure on the right decayed to a muon-neutrino pair, but that is an inessential detail). But they are rare, as you reckon if you compare the height of the curve at 177 GeV with the peak at 80.

The Feynman diagram for the "s-channel" virtual-W production mode of single top is shown on the left below. This diagram must be read "from the left to the right": a quark-antiquark pair fuses, creating the W. The W then turns into a top-antibottom, with the top evidenced in red. And then, the single top produced with the b d

A second way by means of which a single top can be produced (right)

The DZERO analysis
The DZERO collaboration has published several papers on single top production by now, and their tools to sort these rare events out have been tuned very well. Besides the identification of jets containing b-quark decay information, using so-called b-tagging algorithms, DZERO relies on the particular kinematics of the four reconstructed objects, to reduce backgrounds which are due to strong interactions and to regular production of W bosons with additional jets. The discrimination of signal and backgrounds is performed with the help of three separate multi-variate tools: a Bayesian neural network (BNN), a Boosted-Decision-Tree structure (BDT), and a neuroevolution of augmented topologies (NEAT).
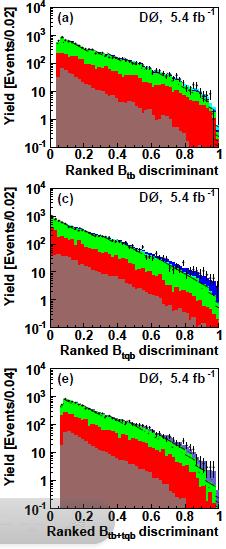
I will not go in the details of these fancy tools, whose basic concept is to sort out the regions of phase space where the signal is most likely to sit. I leave the curious readers to a full reading of the paper, which is well-written. Here, I will just show how the signals look like in this Tevatron endgame, with all the statistics that will likely ever be thrown into these single-top-finding machines.
The figures on the right show the output of the three discriminants for the DZERO data, compared to the expectation of simulations of the various contributing processes. As you can see the small signal shown by the blue histogram is fighting with three main backgrounds: "W plus jets" (in green), top pair production (in red), and QCD multijet production (in brown). But you can also see that the discriminants "push" the blue signal toward the right of the distribution, where it can be evidenced better.
The signal looks tiny in the logarithmic plots, but if one selects events at high values of the discriminants, one realizes that these sub-samples contain a significant amount of signal. This can be seen in the set of plots shown below: the distributions show the transverse mass of the leptonically-decaying W boson (left), the event centrality (center), and the reconstructed top mass (right). I include this latter figure explicitly for one long-time reader of this blog, Tony, who is no doubt interested in it, having in the past advocated the possible presence of a 145-GeV signal in similar distributions. However one must note that the selection of single-top candidates here relies on the knowledge of the top quark mass, so the figure does not allow one to check for top quarks of mass significantly different from 173 GeV...

Conclusions
With the available data DZERO measures the cross section of single top quark production at 0.68+0.38-0.35 pb and 2.86+0.69-0.63 pb for the virtual W and W-gluon fusion processes, respectively; the data is also used to set limits on the value of the Cabibbo-Kobayashi-Maskawa mixing matrix element V_tb, whose strength directly affects the production rates. The limit is set at |V_tb|>0.79, at 95% confidence level. Definitely a very successful analysis by DZERO, while we wait for precision measurements of single-top-quark production by the now unrivaled LHC experiments... Suffices to say that the production of single top at LHC has cross sections twenty times larger, to understand that the study of these processes is now moving to the other side of the Atlantic, as every other hadronic physics topic...
Comments