First of all, the sources. The first one is a Fe-55 emitter that produces very soft x-rays from electron capture - basically, the electron is captured by the nucleus, whereby a proton turns into a neutron with the emission of an electron neutrino. It is the inverse of the well-known beta decay of neutrons, and it can be observed by the spectrometer not because of the final state particles (the neutron cannot be seen, and the neutrino much less so), but because of the distinct x ray (at 5.9 or 6.49 keV, depending on what nuclear configuration is produced at the end) that the electron emits as a shriek of horror as it plunges into the nucleus.
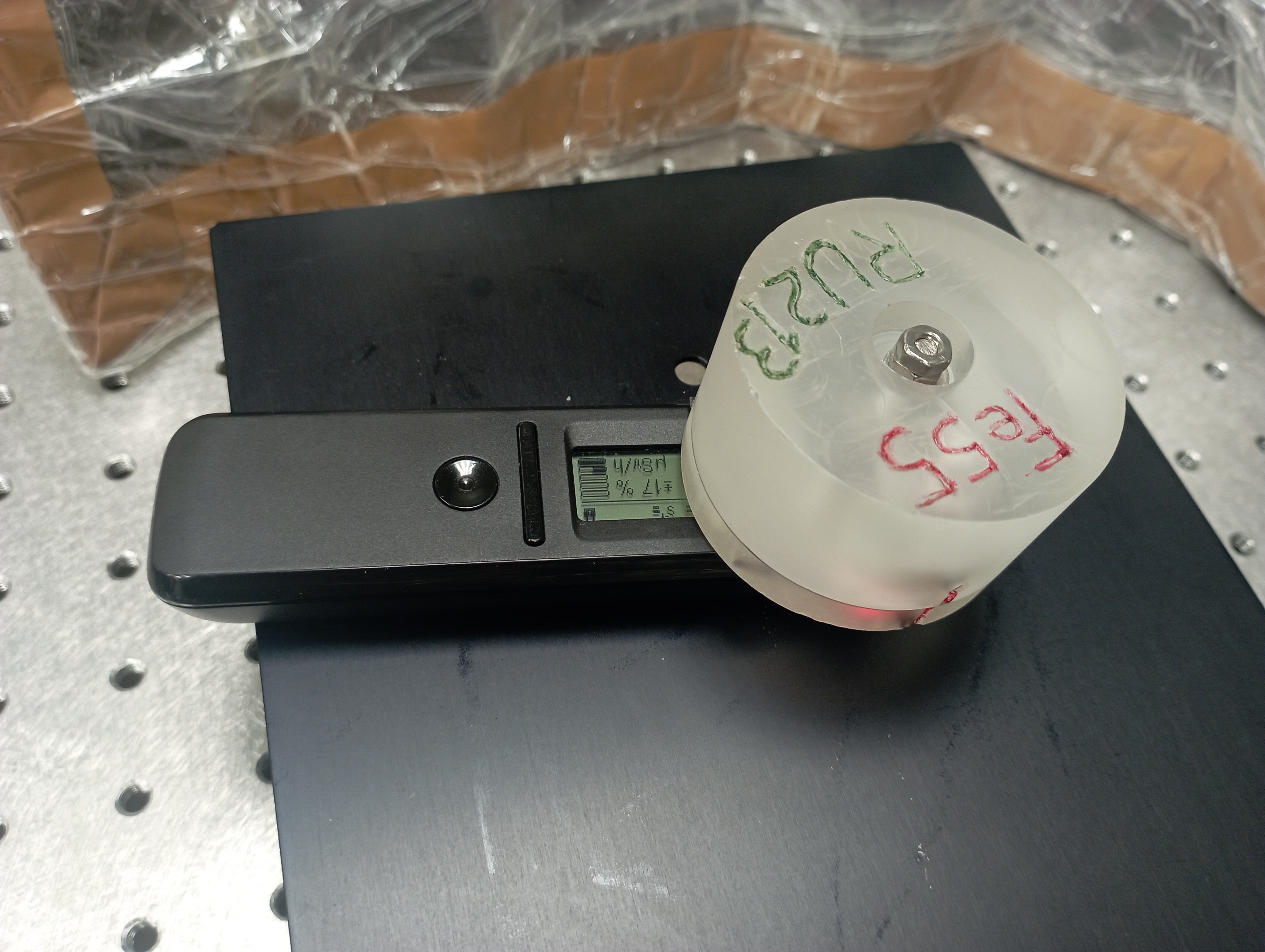
(Above, the Fe-55 source placed over the RadiaCode for the test)
Since the RadiaCode 103 is sensitive to gamma rays above 8 keV, thus nominally above the energy of Fe-55 x-rays, this source presents a challenge for the instrument. However, pileup of several simultaneous x-ray emissions can make the energy release sufficient for detection - or so I believe. The spectrum I acquired looks like this:
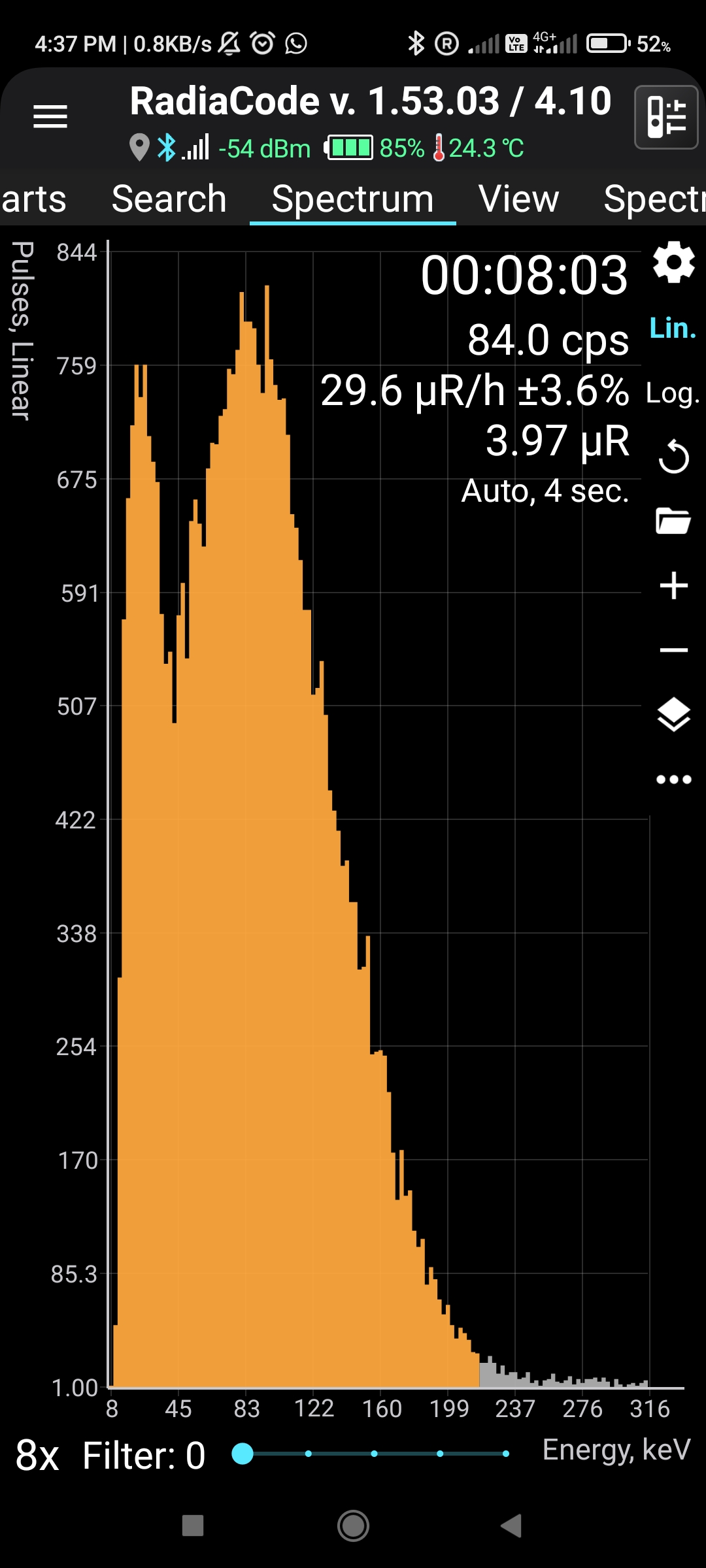
The peak at 20 keV is in a region where the spectrometer has likely not plateau efficiency (as one would expect, and anyway surmise by observing that the "background" distribution peaks at 100 keV or so, an indication that efficiency to softer energy particles could be reduced below that value).
However, the relative energy resolution in that region should be close to the nominal one of the instrument (8.2%), a fact that I have been able to confirm by observing some other isotope lines of very low energy (IIRC it was a source of Ba-133, which produces lines at around 30 keV). So the broad structure at 20 keV is likely due to the superposition of several soft x-rays from electron capture - something that may only happen if the source is strong enough, which it is (I forgot how many megabecquerels it is, but I am led to believe that by placing the RadiaCode 103 sensitive spot on top of the narrow slit exposing the source, I was directing several million photons per second to the NaI crystal).
All in all this test looks rather inconclusive, as it does not allow me to confirm much more than the fact that there is good sensitivity even to sources that emit in a range for which the instrument was not built - provided they are strong enough. So let us move on to the other source, a Sr-90 emitter of beta electrons (the inverse process of that of the Fe-55 one): these are nuclear beta decays whereby a neutron turns into a proton, an electron, and a neutrino. The neutrino escapes unseen, of course, but the electron is duly caught by the instrument, as its interactions with the sodium iodide scintillator produce plenty of light.
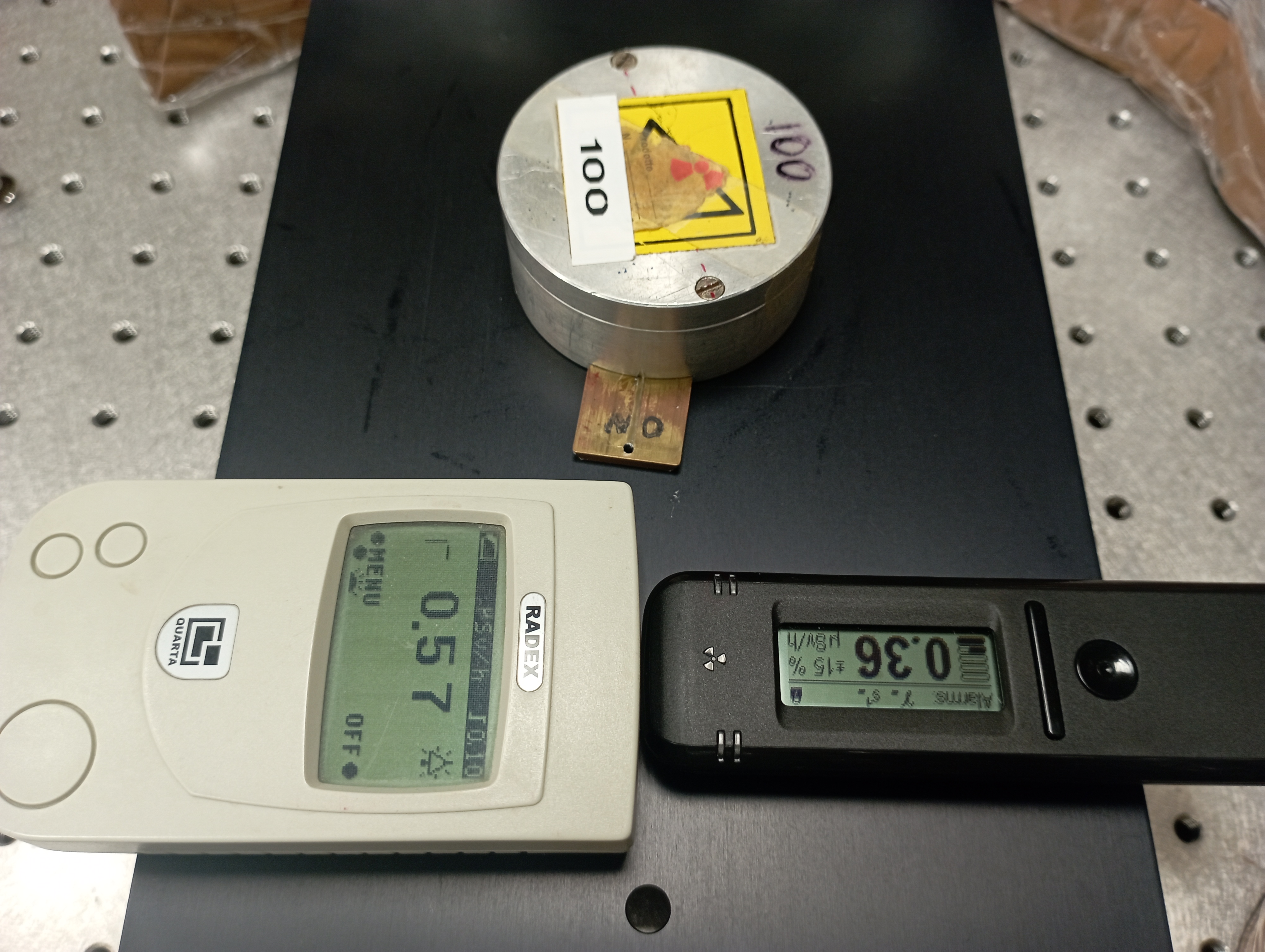
(Above: the Sr-90 source, the RadiaCode 103, and another dosimeter. Note how they detect very little extra radiation, as the source emits gammas only through a hole on the bottom side).
The fact is, though, that since the instrument is calibrated to measure precisely the energy of photons, I do not expect it to return as precise a measurement for electrons. But it does not matter much, either, as the electrons from beta decay are not monochromatic: the presence of three bodies in the final state of neutron decay ensures that we get electrons of all energies, up to a certain "endpoint".
The endpoint for Sr-90 is at 0.546 MeV, where neutron decays produce the highest-energy electrons on one side, and the neutrino and the proton on the opposite side. In this kinematic configuration, the electron is shot out at the maximum kinetic energy. The decay produces a Yttrium nucleus Y-90, which then also undergoes beta decay (turning into Zr-90, a Zirconium nucleus). Now this second beta electron is more energetic because of the fact that the Sr nucleus, which had originally 38 protons and 52 neutrons, decays into a nucleus with 39 protons and 51 neutrons, and this arrangement of nucleons does have less potential energy than the Sr one, but not by much about twice the endpoint energy, 1.1 MeV).
Instead, the Zr nucleus, with 40 protons and 50 neutrons, finds a much more comfortable arrangement of nucleons in a very low energy configuration (indeed, Zr-90 is a stable isotope!), thereby freeing up almost 5 MeV, up to half of which can be transferred to the outgoing electron, in the "endpoint" configuration.
So, to summarize, the source of Sr-90 yields beta electrons with a continuum spectrum of less than 0.546 MeV PLUS a continuum spectrum of less than 2.28 MeV. The two signals overlay to one another, and the instrument produces a spectrum like this:
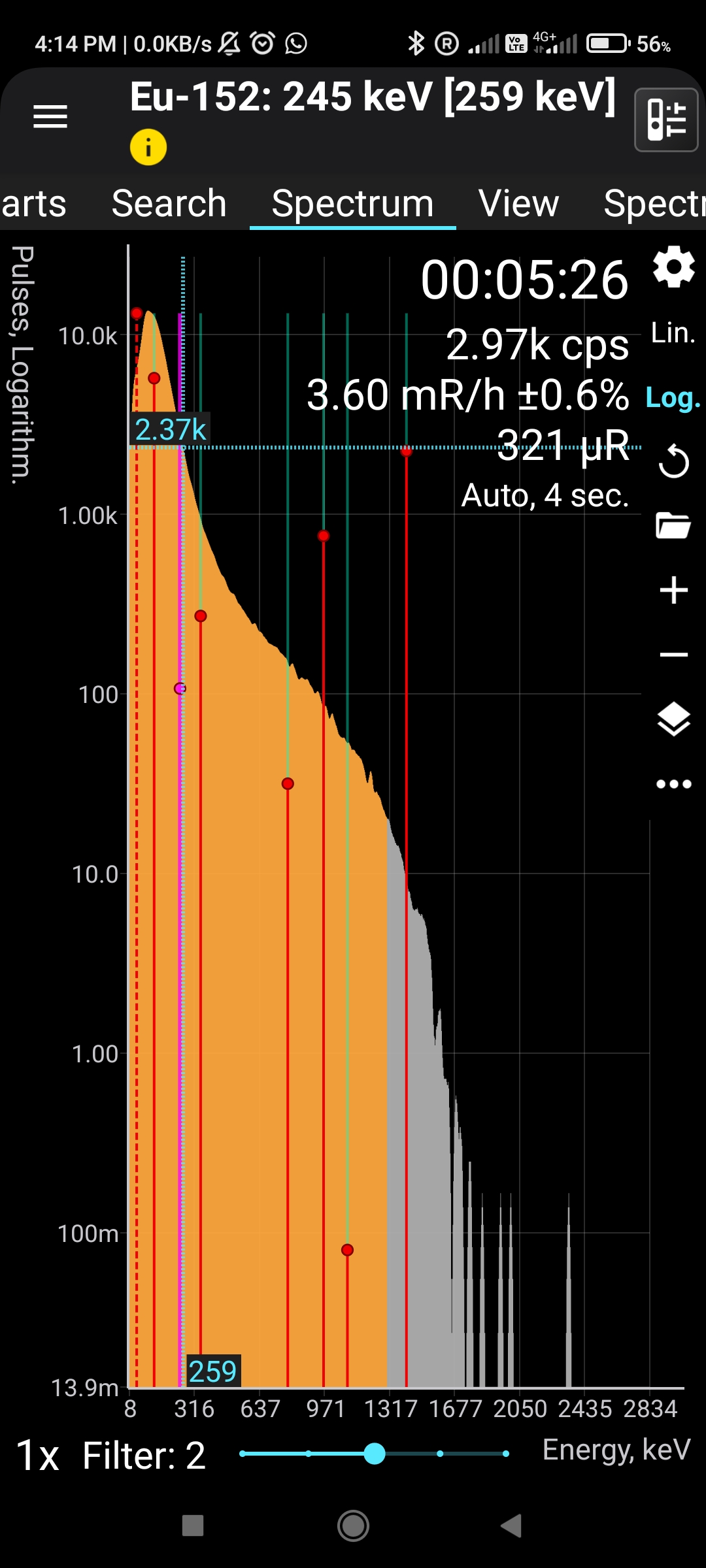
As you can see, indeed there are here two distinct distributions: one peaking at about 100 keV, due to the continuum beta electrons from Strontium; and a second one peaking at about 1.2 MeV (the "hump" visible on the right), and dying out at 2.2 MeV, due to the decays of Yttrium (backgrounds are completely negligible here, due to the high intensity of the source) . It is nice to see that the instrument does not seem to introduce large energy biases to the readings, although it is admittedly a very qualitative observation at this point (I could, if I had the stamina, do a more detailed analysis using the expected distribution of beta electrons, but I am much too busy and lazy to do that!).
As I mentioned in the previous post, at home I have a collection of minerals (I love minerals and have been collecting them for as long as I remember). I well know that some of them are radioactive (two specimens of Autunite and one of Carnotite), and in fact I keep those in a metal box in a drawer, from where almost no gamma ray manages to escape. But I have 500 more samples of various other substances, and I have no idea which one may be radioactive - I only know that none of them is detectably radioactive, with the dosimeter I had until now.
But the RadiaCode 103 is a much more powerful and precise instrument! So I am in the process of testing all the specimens. It is, admittedly, a rather lengthy procedure if done correctly, because we are talking of detecting faint lines in a spectrum that otherwise looks in shape and in total rate like the background from ambient radiation.
The procedure works as follows: you first acquire a long exposure to backgrounds, by placing the instrument somewhere (over my desk) and being careful that no known sources of radiation (e.g. the autunite pieces) are at reach. After 12 hours, I get a rather precise estimate of the spectrum due to ambient radiation (due to radon contamination, cosmic rays, etcetera). Then I am ready to place the sample very close to the RadiaCode 103 - I basically set the device on top of the specimen, such that the sensitive spot of the instrument is directly on top of a crystal of the substance under test.
After a few hours, the spectrum of background + tentative source can be compared to the one of background alone, obtaining something like this:
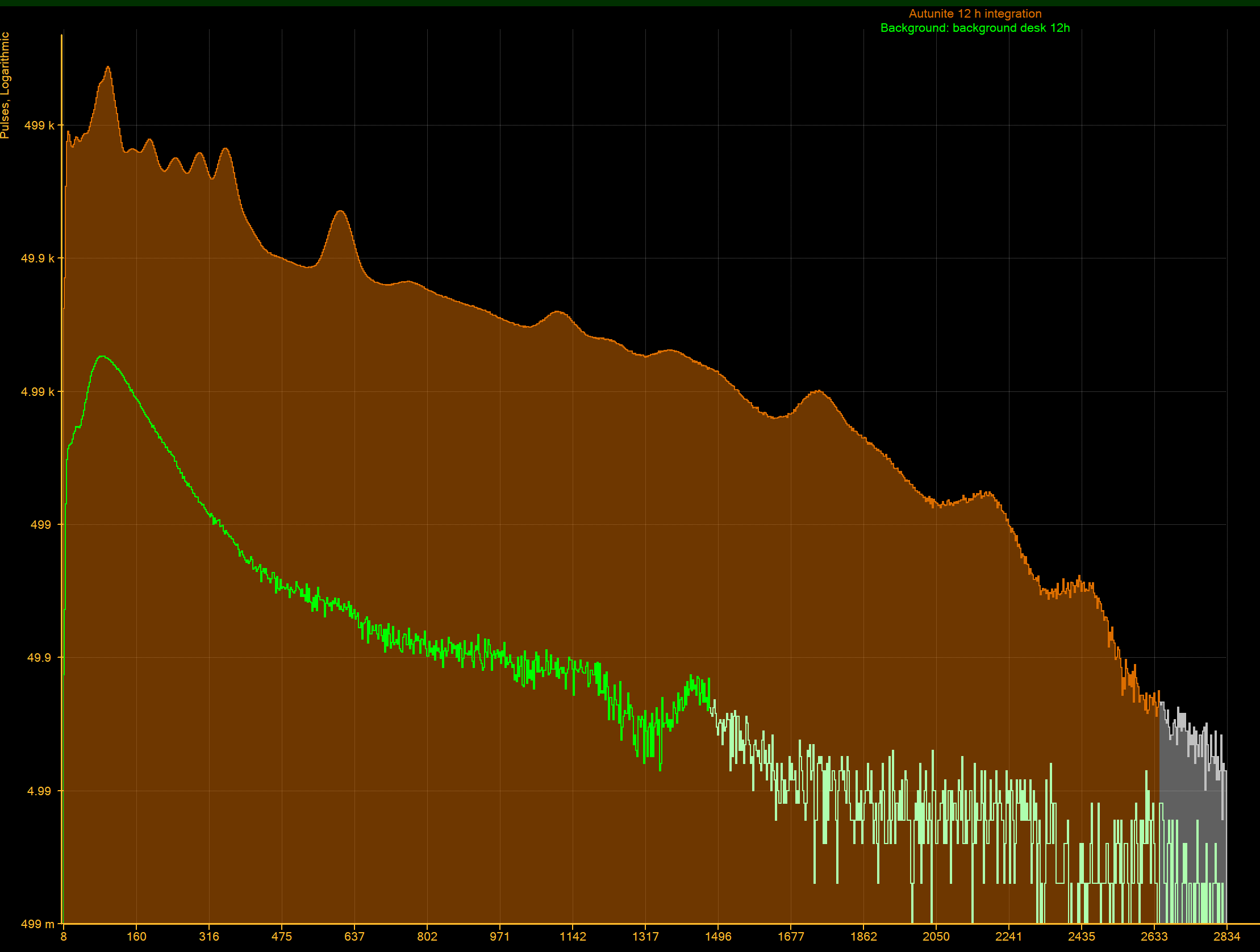
Well, okay, this was an easy target: by acquiring several hours of radiation from the autunite sample, the data you see here shows a wealth of nice isotope peaks... So let us move to harder targets, which have almost undetectably low activity if you only look at the counts per minute.
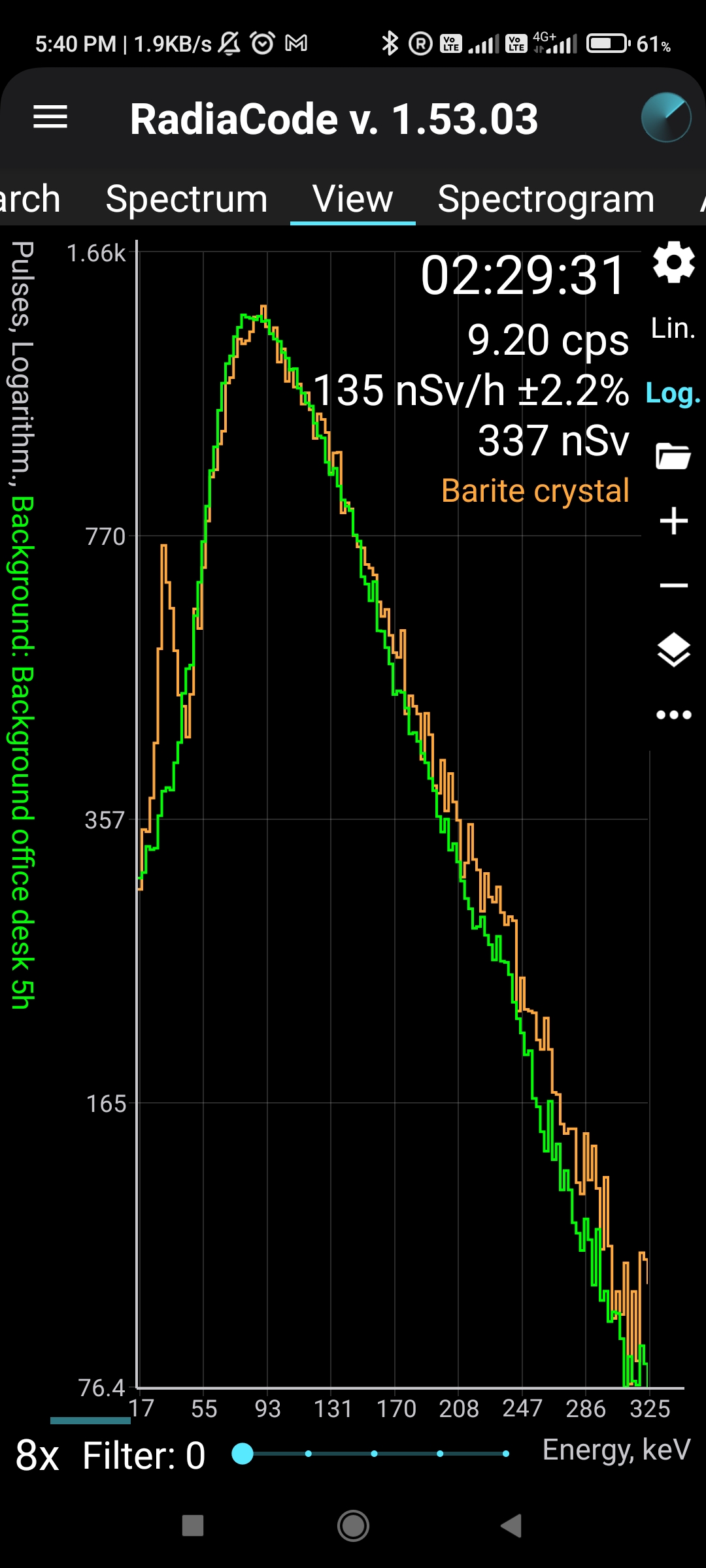
In the case shown above (snapshot taken from the mobile app) we are looking at the spectrum due to a large barite crystal. Barite is a barium sulphate. Barium is a heavy element, not radioactive really, but a crystal will often contain traces of active isotopes. Indeed, as you can see there are indications of the presence of Ba-133 in the sample, as the peak at low energy (between 30 and 36 keV) cannot be due to backgrounds.
A different barite sample from the Czech republic produces quite a different spectrum: in this case, it is easy to recognize, as well as the soft x-rays from Ba-133 on the left, many of the lines produced by isotopes in the Ra-226 chain (including some prominent ones due to Bi-214):
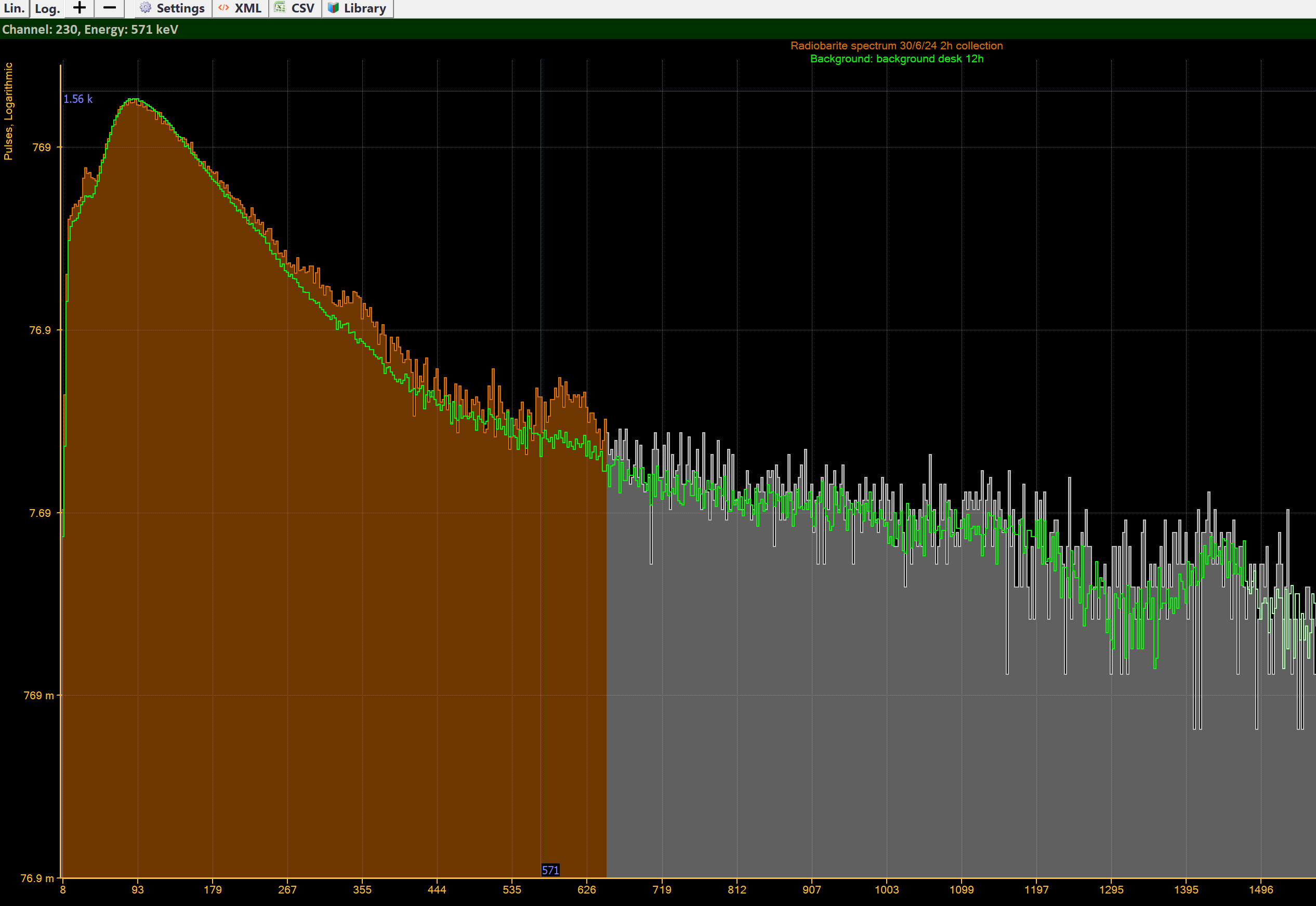
As you clearly see, these are very low radiation samples, and the isotopes can be seen only by acquiring data for a long time. But this, I think, is what makes this instrument so valuable: it has distinct capabilities to produce high-quality data that may provide arbitrarily precise results!
Here (see below), e.g., is a sample of zircon, which I had no idea could be contaminated with uranium until ChatGPT suggested me to test it (along with a few other samples that are in the pipeline of my tests!):
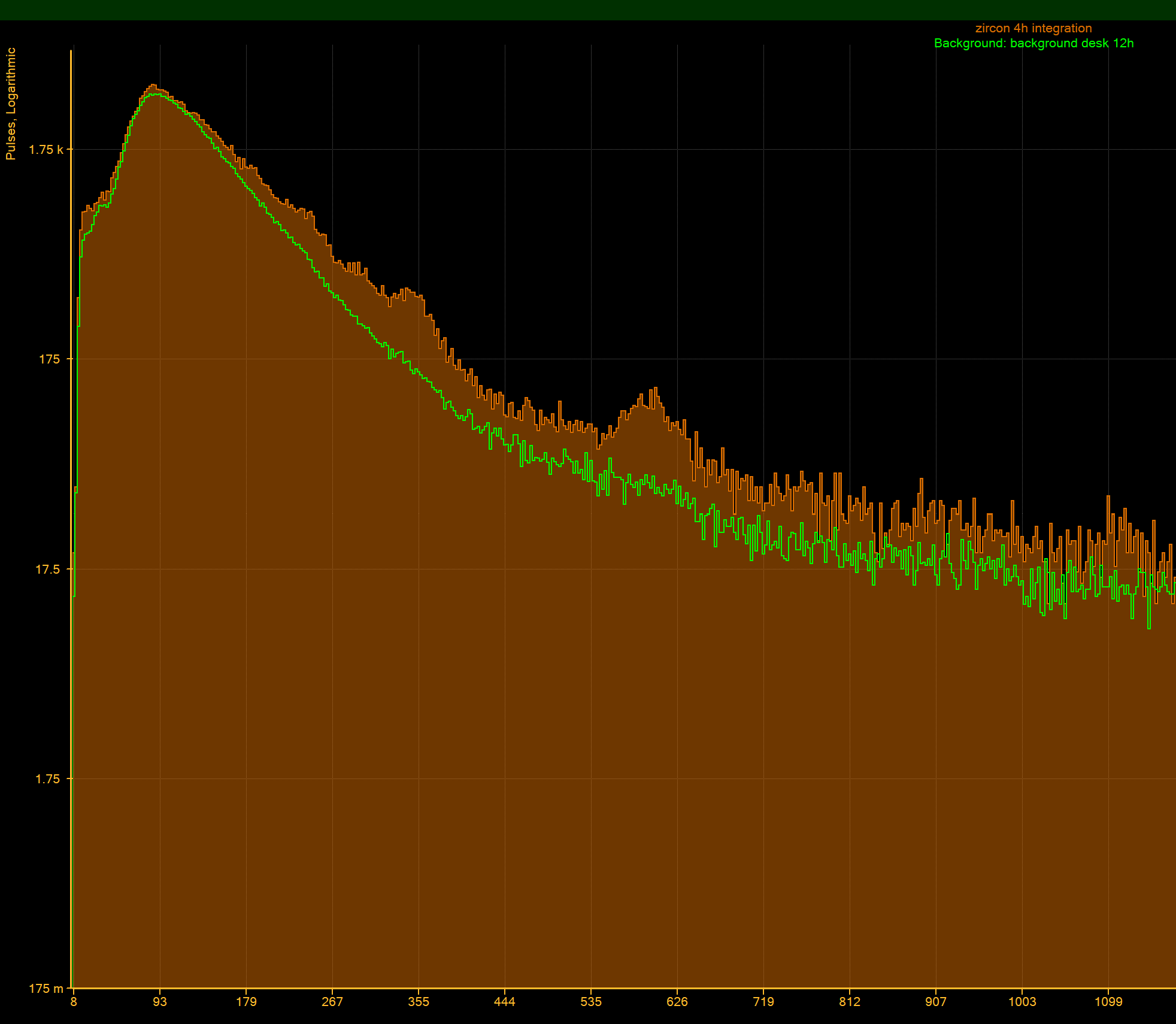
You can clearly see many of the peaks due to the Ra-226 decay chain. The RadiaCode will confirm the location of those peaks if you hover with your pointer or finger on the peaks, but I cannot take snapshots easily with that feature enabled.
So, in summary: the instrument is a powerful little gadget, and it can detect with ease radionuclide contaminations that have activities well below 1 microrem per hour. If you are a forward-thinking person who is also a bit paranoid, you might consider that if a regional nuclear war starts somewhere near you, or if a nuclear plant is leaking stuff, you definitely would like to have one of these with you - but by then, it might not be so easy to get one anymore! So if you have some extra 350$ cash this is definitely a good purchase. My 2 cents!
Comments