The study, titled "Likelihood Functions for Supersymmetric Observables in Frequentist Analyses of the CMSSM and NUHM1" and authored by renowned supersymmetry experts like John Ellis and Sven Heinemeyer, and experimentalists like my CMS colleagues Albert De Roeck and Henning Flacher, had me thinking that Supersymmetry does have an answer for everything, apparently. That, at least, is what one gets from even a careless look at a few of the figures in the paper. But let me get back a few steps and explain what I am talking about, before I am left alone here.
SUSY, a proper daughter of mother Nature
Supersymmetry is an appealing extension of the Standard Model of particle physics, which is both beautiful and naughty. In order to fix a problem of the Standard Model -the unbearable lightness of the Higgs boson- Supersymmetry tries to sell us the existence of dozen new particles yet unseen, fourscore new parameters and then a few, and one additional symmetry principle: for every fermion there is a boson, and for every boson there is a fermion. A beautiful, but broken, new symmetry of mother Nature (another well-known bitch). Broken, because the supersymmetric bodies are all much heavier than their standard counterparts: lest we would have already seen them!
To tell the truth, Susy (that is the nickname by which she goes among those who have entertained themselves with her at least once) is not just easy at claiming new bodies: it also openly displays a pair of additional nice features that make her appealing. On one side, it provides precise high-energy convergence to a common value for the coupling strength of the fundamental interactions. And on the other, it contains a perfect candidate for the unaccounted mass of the Universe among the score of new particles it hypothesizes: the neutralino. Make no mistake: Susy is unification-ready, and just what you need for a big Bang.
The neutralino is the lightest supersymmetric particle. It cannot decay to anything lighter, and so is bound to live forever. It is electrically neutral, so light cannot see it; it is not made of coloured stuff, so ordinary matter hardly stops it; and it is just expected to have the right mass to make the matter balance of our Universe compatible with its evolution after the big bang.
Two words on the study
After the above introduction, it is time to get serious again and discuss the paper. I do not wish to summarize it for you here -it is 34 pages long, and I would be unable to do it in a reasonable amount of time; plus, there is no real reason to do it since the paper is quite readable and you should definitely donwload it from here. All I can do here is to just concentrate on a few of its many interesting results.
To verify the compatibility of Susy with the present experimental status of particle physics and astrophysics, the study considers all electroweak precision data (measurements at the Z peak by LEP and SLC, Tevatron results on the top and W boson masses, neutrino scattering experiments, and then a few), together with the precise measurement of the muon gyromagnetic ratio (a quantity which has a highly predictive power for new physics, since its value would be sizably affected by the existence of new particles circulating inside virtual loops in the scattering diagrams affecting the muon anomaly), B physics observables (which for the same reason have something to say about the existence of those fancy massive new particles), and cosmological constraints. In this sense, it is a really complete account of the inputs we may have at hand to determine whether Susy is just a dream or the girl next door.
Because supersymmetry is not a single theory, but rather a framework of different theories which may display the widest variety of phenomenology depending on the value of a few critical parameters, the study only considers a subset of specific models, ones going by the name of CMSSM and NUHM1. these are particular varieties of the minimal supersymmetric extension of the Standard Model (MSSM), and to explain their details I would need to write more than I am willing to about them here. Suffices to say here that these theories are enough well-defined that they can make definite predictions for some quantities we have a chance of measuring before our retirement.
The authors offer us the results of a fit to all the measured quantities considered in the study, which has the benefit of taking a frequentist approach for the statistical analysis: this frees them from the need of assuming any knowledge of the a priori distribution of some of the parameters, and makes the results insensitive to such assumptions.
The fit is a quite smart one, which investigates with care the full parameter space; all model parameters are varied simultaneously in the sampling of the multidimensional space. The paper explains several technical details that I cannot report here; these give the impression that the work has been done with care, and one has the feeling that the results and their uncertainties may be trusted to be a faithful representation of the current status of our indirect knowledge on Supersymmetry from the available experimental inputs.
An interesting result of the fit is the branching fraction of the Bs meson (a particle made by a strange quark and a anti-bottom one) to muon pairs: within the best solution of the CMSSM this quantity is close to the Standard Model prediction -which is bad, since experimentally we have quite a lot of work ahead before we can measure it if it is so small; on the other hand, it may easily be much larger for the NUHM1, which exposes that theory to a direct proof (or bust) in
a not-so-distant future. In the figure below you see the likelihood functions as a function of Bs branching fraction; the black line shows the Standard Model prediction. You can see that in the NUHM1 model (right) the likelihood has a much flatter minimum extending to several tens in 10^-8.

Maybe the most striking result of the study is the observation that
within the NUHM1 a value of the lightest neutral Higgs boson arises naturally above the lower limit set by the LEP II experiments: in other words, while the Standard Model (and quite a few points of the SUSY parameter space) is hard-pressed to explain why the Higgs boson is heavier than 114.4 GeV, when electroweak data would instead point to smaller values (the latest fits in the LEP electroweak working group page point to
As far as the lightest Higgs boson is concerned, within the CMSSM the best fit produces an estimate of
You can see the results in the figure below, which shows the likelihood functions and their minima as a function of the Higgs mass for the CMSSM (left) and NUHM1 model (right). The curves which are most interesting to me are the dashed ones: they exclude from the fit the knowledge of the lower bound on MH set by the LEP II experiments, and thus show that the NUHM1 model does prefer a mass above the crucial 114.4 GeV divide.
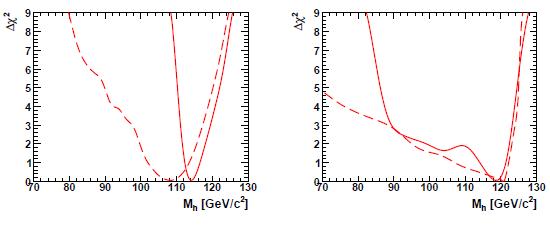
Also worth mentioning here is that for the mass of the lightest neutralino, the best fit value turns out to have a sharp minimum at about 120 GeV in both considered models: this is not a surprise, but it is again interesting from an experimental standpoint, since such mass values may be accessible in the near future.
The paper contains several plots like the ones shown above, describing the likely values of many of the important parameters of the theories considered. If you are at all interested in knowing what is the most likely mass value of your favorite -ino particle, your curiosity will be satiated.
As for me, I find it remarkable that Supersymmetry (or at least a few points of its hundred-dimensional parameter space) keeps standing head and shoulder above the surge of experimental results coming out of the Tevatron, which stubbornly insists finding everything in agreement with the Standard Model and no trace of sparticles around. In principle, in three months time the start-up of LHC might allow us to discover Supersymmetry in the matter of a few weeks, or even days of running. Well, make it a few months. Reality, I am told, is usually different. Life is tough, Nature is a bitch, and Susy is presently hiding in the dark. Stay tuned.
Comments